Bạn có nhu cầu theo dõi các trận đấu bóng đá mà không phải trả phí và bị quấy rối bởi quảng cáo? Bạn đang gặp khó khăn khi tìm kiếm một trang web chất lượng để xem bóng đá? Giờ đây, bạn không cần phải tìm kiếm đâu xa, chúng tôi sẽ giới thiệu cho bạn biết một nền tảng xem bóng đá chất lượng mang tên Socolive.
Giới thiệu sơ về kênh trực tiếp bóng đá Socolive
Socolive TV, một trang web xem bóng đá trực tuyến được rất nhiều người quan tâm, nhưng ít ai hiểu rõ về nguồn gốc và lý do chọn tên của nó. Chúng tôi sẽ làm sáng tỏ những thắc mắc đó trong phần nội dung sau đây.
Socolive là gì?
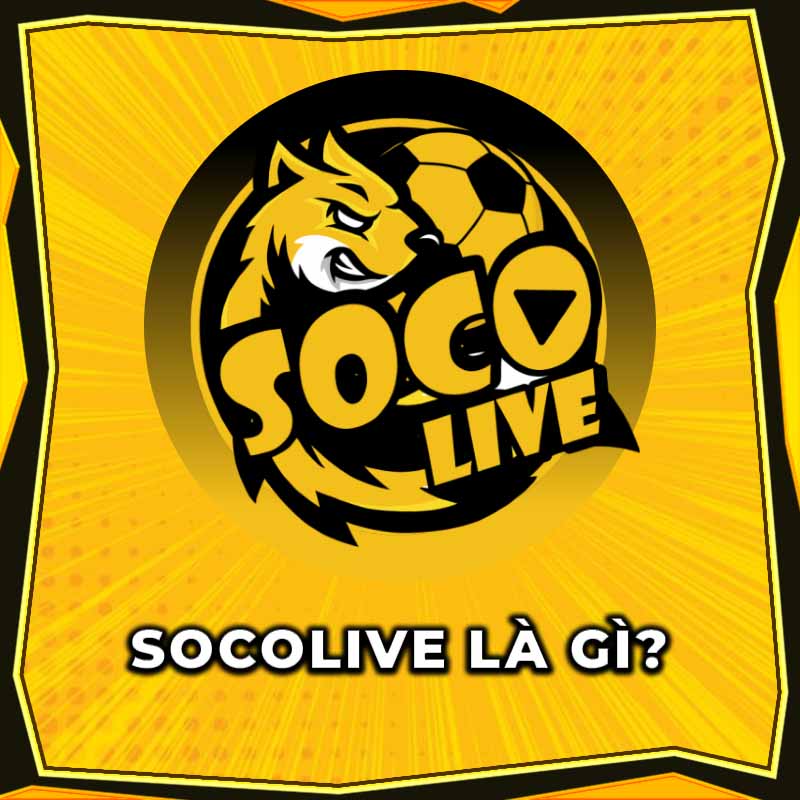
Socolive là một nền tảng trực tiếp các trận đấu bóng đá với nhiều tiện ích đi kèm. Với cam kết mang đến tất cả các trận đấu trên thế giới đến với người xem Việt Nam, trực tiếp bóng đá Socolive phát sóng hết thảy những giải đấu lớn nhỏ dù là giải quốc tế hay nội địa.
Ngay cả khi bạn không thể thưởng thức trực tiếp, Socolive TV vẫn là điểm hẹn lý tưởng để bạn có thể xem lại những trận đã diễn ra trước đó. Ngoài ra bạn còn có cơ hội cập nhật đầy đủ thông tin thể thao xoay quanh thế giới bóng đá. Chúng tôi tin rằng truc tiep socolive sẽ thỏa mãn niềm đam mê bóng đá của bạn.
Giải thích nguồn gốc tên gọi Socolive
Có lẽ nhiều người sẽ muốn tìm hiểu về nguồn gốc của cái tên “Socolive”. Sỡ dĩ cái tên soccolive là một sự kết hợp khéo léo giữa “Soccer” (bóng đá) và “live” (trực tiếp). Dù tên gọi có vẻ đơn giản, nhưng trong tên gọi đã nói lên được mục đích hoạt động của trang web.
Từ cái tên chúng ta có thể hiểu đây là một nền tảng trực tiếp đặc biệt dành cho các sự kiện bóng đá. Với tên gọi này, mỗi khi nhắc đến m.socolive, chúng ta ngay lập tức liên tưởng đến một trang web có nhiều trận đấu bóng đá hàng đầu trên thế giới.
Quá trình phát triển
Thực tế bóng đá đã trở thành một món ăn tinh thần không thể thiếu trong cộng đồng người hâm mộ thể thao ở Việt Nam. Socolive được nhiều người xem như một ứng dụng xem trực tiếp bóng đá miễn phí chất lượng hàng đầu. Người xem sẽ trải nghiệm xem bóng đá với chất lượng hình ảnh và bình luận chuyên nghiệp.
Ra mắt chính thức vào ngày 19/11/2021, sau 2 năm hoạt động trang web không ngừng mở rộng và đạt được thành công bằng cách thâu tóm 4 trang bình luận bóng đá hàng đầu khác. Từ giai đoạn này truc tiep bong da socolive càng được nhiều người biết đến hơn khi nắm trong tay đội ngũ nhân sự chuyên nghiệp với quy mô lớn.
Những tiện ích bạn được trải nghiệm với Socolive TV
Không phải kênh cũng có thể ghi điểm như trực tiếp bóng đá Socolive, điều này không phải là do sự ngẫu nhiên. Để đạt được danh tiếng như hiện nay, socolive 1 đã đầu tư rất nhiều vào việc cải thiện các dịch vụ của mình. Hãy cùng khám phá những tính năng nổi bật mà trang web đã phát triển để tỏa sáng trong lòng khán giả.
Xem bóng đá trực tiếp
Socolive phát sóng miễn phí cho tất cả các giải đấu bóng đá có bản quyền trên toàn thế giới, và tất cả đều được trải nghiệm với chất lượng hình ảnh tốt nhất. Khi xem bóng đá trên trang web này, bạn có thể tận hưởng mọi trận đấu quan trọng mà không phải trả bất kỳ chi phí nào.
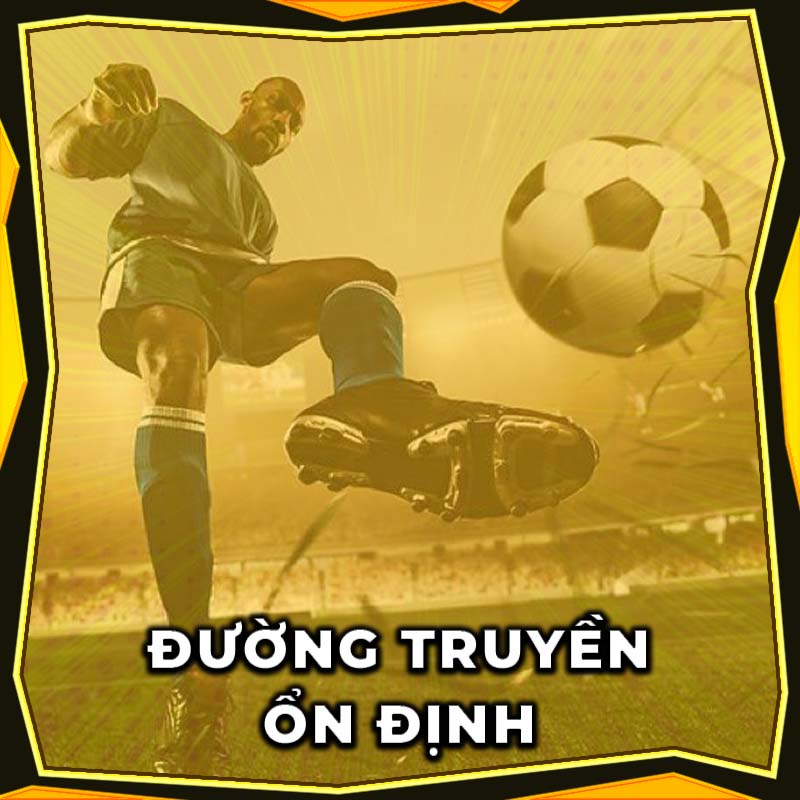
Hiện nay ngoài sôclive thì còn có nhiều nền tảng xem bóng đá khác như K+, VTVcab…, đây là những kênh xem bóng đá chất lượng nhưng yêu cầu trả phí dịch vụ. Nên nếu bạn muốn xem bóng đá có bản quyền nhưng miễn phí thì chỉ có Socolive làm được.
Livescore
Ngoài việc cung cấp dịch vụ trực tiếp xem bóng đá, kênh bóng đá tích hợp tính năng hiển thị tỷ số trực tiếp của các trận đấu. Người dùng có thể theo dõi tiến triển của các trận đấu đang diễn ra và cập nhật tỷ số một cách nhanh chóng và chính xác. Với tính năng này đảm bảo rằng người hâm mộ sẽ luôn được thông tin về tình hình trận đấu mà không cần phải xem trực tiếp.
BXH
Socolive TV cung cấp đầy đủ về bảng xếp hạng của các đội bóng. Mỗi khi một trận đấu kết thúc, bảng xếp hạng sẽ được cập nhật ngay lập tức để người hâm mộ cái nhìn chính xác về vị trí hiện tại của các đội.
Khác biệt giữa Bảng xếp hạng và Livescore trên Soco live là Livescore ghi nhận kết quả bóng đá theo thời gian thực, thích hợp cho những trận đấu đang diễn ra. Điều này đồng nghĩa với việc người dùng có thể cập nhật ngay lập tức về tình hình thi đấu và kết quả dựa trên từng thời điểm trong trận đấu.
Trong khi đó, bảng xếp hạng cung cấp cái nhìn chi tiết về thứ hạng của các đội bóng, tạo nên một cơ sở dữ liệu đáng tin cậy để thực hiện các so sánh và đánh giá hiệu suất của các đội theo thời gian. Sự kết hợp giữa Livescore và bảng xếp hạng trên socolive news đảm bảo rằng người hâm mộ có mọi thông tin cần thiết để theo dõi và đánh giá sự phát triển của giải đấu.
Bản tin bóng đá
Với trực tiếp bóng đá Socolive, việc kiểm tra thông tin liên quan đến lịch thi đấu, tỷ số, địa điểm và thời gian của các trận đấu không còn khó khăn nữa Kênh bóng đá này cung cấp đầy đủ về các giải đấu hàng đầu toàn cầu, bao gồm cả bảng xếp hạng và thành tích của những đội bóng nổi tiếng.
Ngoài ra, trang web chia sẻ chi tiết về thông tin bên lề như về tình hình cá nhân cẩu các siêu sao, chuyện chuyển nhượng, HLV đội bóng, sinh hoạt CLB…
Lịch thi đấu
Lịch thi đấu của các đội tuyển bóng đá trên toàn thế giới được cập nhật liên tục trên trang web socolive1. Chúng tôi hứa hẹn nền tảng này sẽ giúp mọi người yên tâm sẽ không bỏ lỡ bất kỳ trận đấu bóng đá nào. Lịch thi đấu được tổ chức một cách có tổ chức trên trang web. Người dùng dễ dàng theo dõi thông tin về thời gian và địa điểm của từng trận đấu.
Highlight bóng đá
Nếu bạn quá bận rộn để theo dõi toàn bộ trận đấu yêu thích, việc sử dụng tính năng xem Highlight trên kênh là một lựa chọn lý tưởng. Với tính năng này sẽ tập trung vào việc hiển thị video highlight, đưa ra những tình huống nổi bật như các pha ghi bàn, những pha phá bóng của thủ môn, những cú xử lý kỹ thuật xuất sắc, hoặc các tình huống pressing giao tranh cực gắt.
Ngoài ra, highlight có phần bình luận chuyên môn để người dùng có thể hiểu rõ hơn về diễn biến của trận đấu mà họ quan tâm.
Xem bóng đá có BLV
Bạn sẽ có một trải nghiệm hấp dẫn với bình luận viên tiếng Việt trong mỗi trận đấu. Bình luận viên mang lại sự sống động và khiến trận đấu có tính tương tác với khán giả hơn. Những lời bình phẩm giúp người xem hiểu rõ hơn về chiến thuật và tình hình trận đấu. Hay hơn nữa là BLV có thể chia sẻ cảm xúc, ý kiến và đánh giá về từng động thái trên sân cỏ giúp người xem có thêm kiến thức về bóng đá.
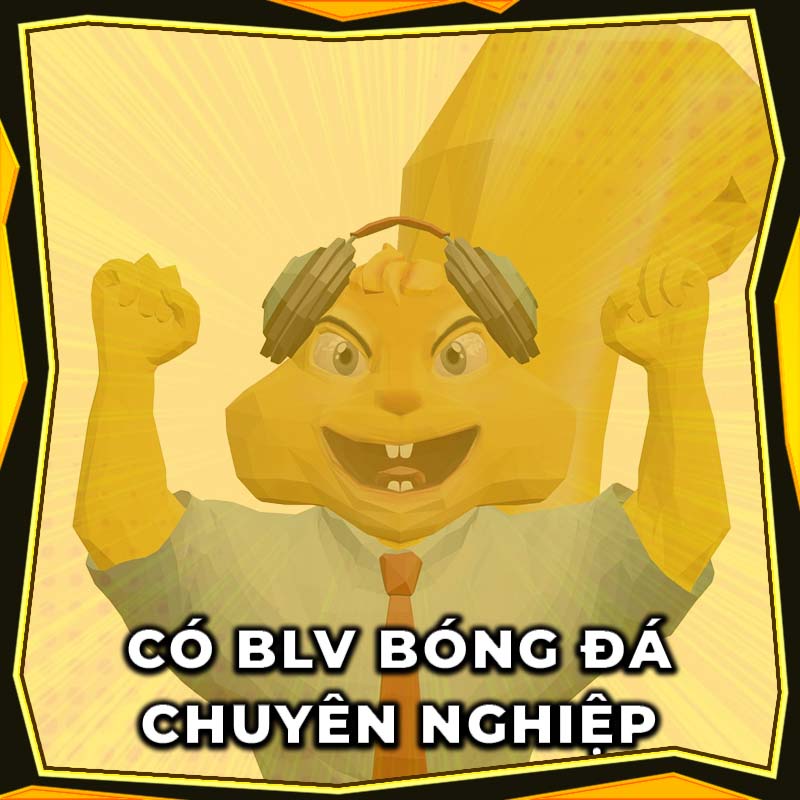
Quyền lợi bạn nhận được khi sử dụng Socolive trực tiếp
Socolive 2 nhanh chóng trở thành một địa chỉ xem bóng đá hấp dẫn trong khoảng thời gian ngắn. Việc trang web nhanh chóng được nhiều người chú ý cũng có lý do của nó. Đây là ưu điểm của socolive vip:
Xem bóng đá chất lượng
Socolive xây dựng hệ thống âm thanh sống động bằng cách thu thanh trực tiếp tại hiện trường. Người xem có thể trải nghiệm âm thanh chân thực và sống động, như thể họ đang ngồi trên khán đài trực tiếp. Đội ngũ kỹ thuật của socolive 3 không ngừng phát triển công nghệ âm thanh, đặt mục tiêu là cung cấp chất lượng tốt nhất cho người xem.
Khi theo dõi trực tiếp các trận đấu bóng đá qua socolive liefie, người xem sẽ bị cuốn hút trong không khí âm thanh sống động từ sân vận động. Trải nghiệm này không chỉ dừng lại ở sự chất lượng từ âm thanh, hình ảnh của trực tiếp bóng đá Socolive cũng rất chân thật. Sự kết hợp hài hòa giữa âm thanh và hình ảnh sẽ giúp bạn có một buổi xem bóng đá chất lượng.
Xem bóng đá mọi nơi
Khi bạn đang ở bất kỳ đâu, chỉ cần một chiếc điện thoại thông minh kết nối internet bạn sẽ dễ dàng truy cập socolive2 để theo dõi trực tiếp các trận đấu bóng đá nóng hổi. Kênh bóng đá này mang lại sự thuận tiện để giúp người xem thưởng thức những trận cầu đỉnh cao không giới hạn.
Ngay cả khi bạn không có cơ hội xem bóng đá trực tuyến socolive, bạn không cần phải quan tâm đến vấn đề đó. Bạn có thể dễ dàng tìm đến dịch vụ lưu trữ để xem lại những trận đấu đã diễn ra. Nếu bạn gặp khó khăn trong việc lấy link, đội ngũ nhân viên chăm sóc khách hàng sẽ sẵn lòng hỗ trợ và cung cấp đường link xem cho bạn. Chúng tôi đảm bảo rằng bạn sẽ không bỏ lỡ bất kỳ khoảnh khắc nào trên sân cỏ.
Không tính phí
Socolive TV biết rằng đối tượng chủ yếu thường là những người có thu nhập thấp. Trong khi đó những người có thu nhập cao thường ưa chuộng các dịch vụ truyền hình trả phí để theo dõi các trận đấu.
Do đó, từ khi bắt đầu hoạt động Socolive đã quyết định không hướng đến mục tiêu vì lợi nhuận. Nói một cách khác, khi bạn trải nghiệm dịch vụ tại đây, không có bất kỳ chi phí nào phải thanh toán.
Hạn chế quảng cáo
Socolive trực tiếp cam kết giảm thiểu sự xuất hiện của quảng cáo nhằm tạo ra trải nghiệm xem bóng đá trực tuyến tốt nhất cho người dùng. Nền tảng nhận thức rõ ràng về việc quảng cáo có thể gây gián đoạn và làm phiền lòng người xem truc tiep bong da soc, đặc biệt là khi thưởng thức các trận đấu quan trọng. Do đó sẽ không có bất kì quảng cáo nào xuất hiện khi bạn đang xem bóng đá socolive.
Tuy nhiên, để duy trì hoạt động và tiếp tục cung cấp dịch vụ miễn phí cho người dùng, bóng đá socolive cần một nguồn thu nhập từ quảng cáo. Do đó, trang web này cũng cần liên kết với các đối tác nhưng Socolive 6 sẽ đảm bảo rằng quảng cáo sẽ được hiển thị một cách hợp lý, không làm gián đoạn trải nghiệm xem bóng đá của bạn.
Đường truyền ổn định
Kênh trực tiếp bóng đá này có tốc độ đường truyền vô cùng ổn định. Socolive đã tập trung đầu tư tiền của vào việc xây dựng hệ thống máy chủ và cơ sở hạ tầng tiên tiến để đảm bảo rằng mọi người có thể trải nghiệm truyền hình trực tuyến mà không gặp tình trạng giật lag.
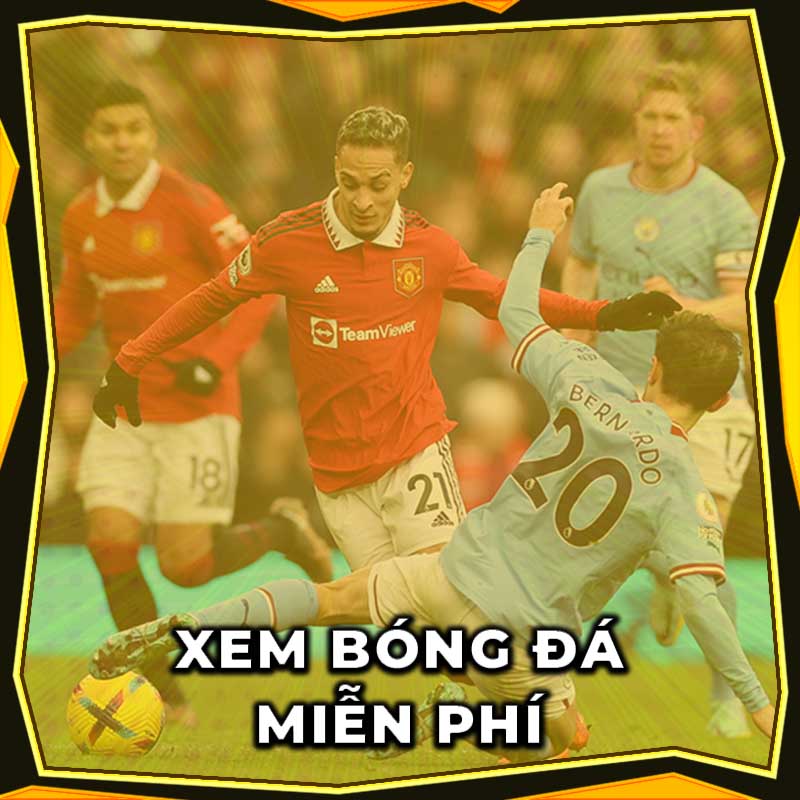
Bên cạnh đó, đội ngũ kiểm duyệt cũng không ngừng kiểm tra và nâng cấp hệ thống để đảm bảo tốc độ truy cập nhanh chóng với chất lượng tốt nhất. Đội ngũ kỹ thuật viên tại socolive so luôn sẵn sàng giải quyết mọi vấn đề kỹ thuật, đảm bảo rằng không có ai phải khó chịu với những khó khăn không đáng có liên quan đến vấn đề công nghệ.
Nhiều đường link xem bong da socolive, hạn chế giật lag
Socolive cam kết sẽ giải quyết vấn đề giật lag một cách chủ động thông qua việc cung cấp nhiều đường link dự phòng cho mỗi trận đấu. Khi số người truy cập tăng quá nhiều trên một đường link chính là https://advancinggreenchemistry.org/ có thể dẫn đến tình trạng giật lag và làm ảnh hưởng đến trải nghiệm xem trực tiếp bóng đá hôm nay socolive của người hâm mộ.
Nền tảng đã triển khai chiến lược này để giảm áp lực và đảm bảo khả năng truy cập mượt mà.
Mỗi trận đấu trên trang web của trực tiếp bóng đá Socolive sẽ được cung cấp với một đường link chính và hai đường link dự phòng. Nếu đường link chính trở nên quá tải và người xem gặp khó khăn khi truy cập, thì họ có thể dễ dàng chuyển sang sử dụng một trong hai đường link dự phòng khác.
Việc trực tiếp socolive tại đường link dự phòng sẽ không làm ảnh hưởng đến chất lượng trải nghiệm. Bit.ly/socolive cam kết rằng cả ba đường link đều đảm bảo chất lượng hình ảnh và âm thanh tương đương, mục đích của 2 đường link phụ chỉ để giảm tải số người tham gia quá nhiều tại 1 đường link.
Tương thích đa thiết bị
Socolive TV hỗ trợ người dùng xem bóng đá trực tuyến trên nhiều thiết bị khác nhau. Khác biệt hoàn toàn với nhiều trang web khác, socolive link không hạn chế người xem chỉ vào việc sử dụng trên TV hoặc laptop mà còn hỗ trợ một loạt các loại thiết bị khác nhau. Điều này không chỉ mang lại sự linh hoạt mà còn tạo ra sự tiện lợi cho khách hàng, giúp họ có thể tận hưởng trải nghiệm xem bóng đá một cách chủ động mọi lúc, mọi nơi.
Khả năng tương thích với nhiều thiết bị, từ điện thoại di động và máy tính bảng đến smart TV làm cho Socolive trở thành điểm đến lý tưởng cho những ai muốn xem trận đấu bất kỳ khi nào và ở đâu.
FAQS xung quanh chủ đề Socolive TV
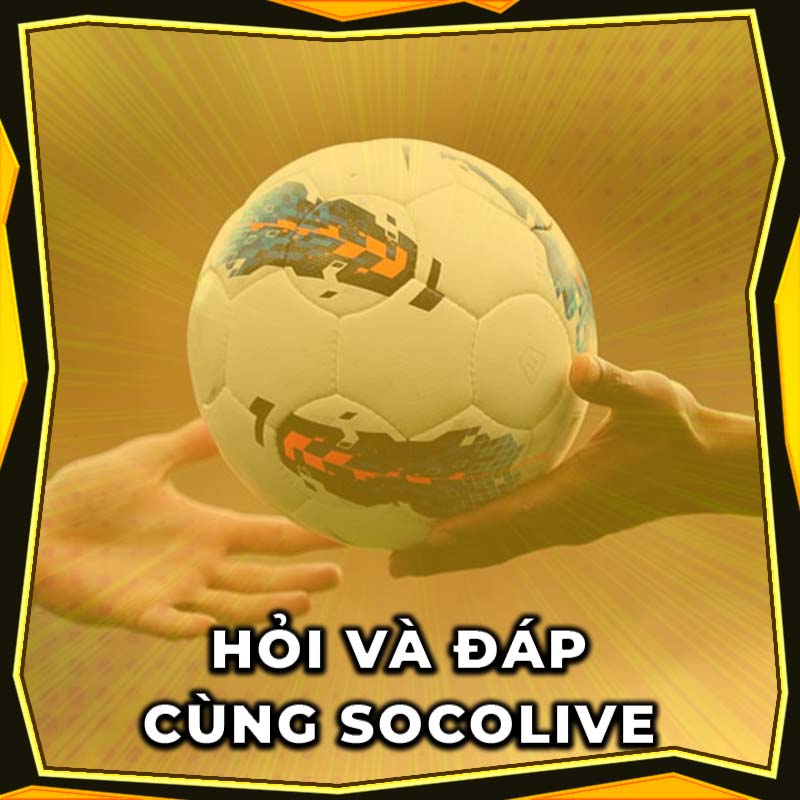
Nếu đây là lần đầu tiên bạn tham gia trải nghiệm xem bóng đá trực tuyến trên socolive 1 tv, có thể có nhiều thắc mắc mà bạn muốn được giải đáp. Dưới đây, chúng tôi sẽ mang đến những thông tin chi tiết để giúp bạn nhanh chóng làm quen với trang web này:
Truc tiep Socolive có thực hiện tính phí với người dùng?
Không! Trực tiếp bóng đá Socolive hiện đang hoạt động theo mô hình không thu phí như một tổ chức phi lợi nhuận. Do đó, tất cả người dùng đều có khả năng truy cập trang web và tận hưởng các dịch vụ mà không phải trả bất kỳ chi phí nào.
Khi truy cập Socolive TV có bị chặn như nhiều web khác?
Bởi Socolive là trang web hợp pháp không liên quan đến cá cược nên sẽ không bị nhà mạng chặn truy cập. Tuy nhiên bitly/socolive có thể phải đối mặt với vấn đề chặn truy cập trong hai tình huống khác nhau.
- Thứ nhất, nếu có quá nhiều người truy cập đồng thời, có thể xảy ra tình trạng quá tải và gây sập hệ thống. Tuy nhiên, tình trạng này thường chỉ xuất hiện trong một khoảng thời gian ngắn và sẽ được khắc phục ngay sau đó.
- Thứ hai, trang web có thể bị chặn khi đang thực hiện các hoạt động bảo trì để sửa lỗi hoặc cải thiện hiệu suất hệ thống. Trong tình huống này, người dùng chỉ cần đợi một thời gian ngắn sau đó thoát ra và truy cập lại để đảm bảo rằng việc truy cập sẽ được thực hiện thành công.
Cả hai trường hợp trên rất hiếm khi xuất hiện trên trang web. Với những trường hợp bất khả kháng như bảo trì trang web thì socolive live sẽ chủ động thông báo trước và đưa ra link chuyển hướng để người chơi sử dụng trong lúc thực hiện.
Socolive có phải là thương hiệu liên kết nhà cái không?
Socolive bóng đá cam kết không hợp tác hoặc liên kết với bất kỳ nhà cái cá cược nào. Mục tiêu của trang web hướng đến là tạo ra một môi trường lành mạnh cho cộng đồng người hâm mộ bóng đá. Socolive football sẽ không tham gia vào bất kỳ hoạt động cá cược nào và tập trung hoàn toàn vào việc cung cấp một nền tảng xem bóng đá trực tuyến chất lượng và an toàn cho bạn.
Tôi không có tài khoản, vậy có cần đăng ký để xem bóng đá không?
Không, bạn không cần phải đăng ký tài khoản để thưởng thức trực tiếp bóng đá trên soco. Trong thời đại ngày nay, nhiều kênh trực tiếp bóng đá yêu cầu người dùng đăng ký tài khoản thường gây phiền toái và tốn thời gian. Socolive đã nhận ra vấn đề này và giảm bớt yêu cầu đăng ký tài khoản.
Việc không yêu cầu đăng ký tài khoản đã giải quyết nhiều vấn đề, đặc biệt là đối với những người chỉ muốn xem bong da socolive cơ bản hoặc những người muốn trải nghiệm dịch vụ mà không muốn mất công đăng ký tài khoản.
Lời kết
Ở đây, chúng tôi đã chia sẻ với độc giả những thông tin hữu ích nhất về socolive. Đây là một website bóng đá trực tuyến hữu ích để thỏa mãn niềm đam mê thể thao của bạn. Khi truy cập trang web advancinggreenchemistry.org bạn sẽ được biết đến nhiều hơn về những kiến thức và kinh nghiệm về bóng đá. Nếu bạn muốn biết nhiều hơn về các thông tin bóng đá hữu ích ngoài việc xem đá bóng, hãy đón đọc các bài viết liên quan của kênh tại chuyên mục tin bóng đá của Socolive nhé!